|
|
|
The Chemist Volume 94 | Number 1 |

|
|
Formulation and Sustained Release Verapamil Hydrochloride Tablets
|
|
Abstract: Verapamil hydrochloride effervescent tablets are the principal focus of this investigation. Material & Methods: Verapamil hydrochloride floating tablets were made using the direct compression method. HPMC-K15M, karaya gum, sodium bicarbonate, and diluents were homogenized for ten minutes before adding magnesium stearate to each tablet formulation. Each tablet had a total weight of 300 mg. HPMC was utilized in the range of 20- 40 mg, and karaya gum was used in the 40-90 mg range. We used a mortar and pestle for kneading the powder combination for another 5 minutes. A Rimek rotating tablet machine was used to compress the mixture into tablet form. The formulations were tested using a variety of criteria following their production. Results & Discussion: The tablet formulation friability range was between 0.3 ± 0.0064 and 0.59 ± 0.0077%. The manufactured tablet formulation's weight fluctuation is within USP guidelines. The range of thickness was discovered to be 4.1 ± 0.48 to 4.2 ± 0.76 mm. The assay for drug content range was between 96.52 ± 0.35 and 102.13 ± 0.53%. For B1, B5, B6, B9, and B10 at 12 hours, more than 75% of the medication was released. B1 showed a maximum of 30% drug release in the first hour and a steady release for up to 12 hours. One possible explanation for B8's low drug release is the creation of a thick gel barrier on top of the tablet.
Key Words: Effervescent, Verapamil, bioequivalence, FDDS, floating tablet
|
|
|
Introduction
Floating drug delivery systems "FDDS" or hydrodynamically controlled systems are low-density systems to remain buoyant in the stomach for a long time without affecting the rate at which the stomach empties. While floating on the gastric contents, the drug is gradually taken from the body at the desired rate. Immediately after taking the drug, the stomach's remaining systems are flushed out. A longer period of stomach retention and improved control over plasma medication concentrations are achieved as a result [1]. Additionally, a certain level of floating force (F) is needed to maintain the dose form stable on the surface of the meal so that the buoyancy retention principle can be appropriately applied. Buoyant systems can be made using granules, powders, capsules, tablets, laminated films, and hollow microspheres, among other things [2-15].
Materials & Methods
Verapamil hydrochloride floating tablets were made using the direct compression method. We blended HPMC-K15M, karaya gum, sodium bicarbonate, and diluents for ten minutes before adding magnesium stearate to each tablet formulation, which included the medicine. Table 1 shows the formulation chart of effervescent floating Verapamil hydrochloride tablets. Each tablet had a total weight of 300 mg. HPMC was utilized in the range of 20-40 mg, and karaya gum was used in the 40-90 mg range. A mortar and pestle were used to knead the powder combination for another 5 minutes [16,17].
Weight variation test
The average weight of 20 tablets from each formulation is calculated by weighing each tablet individually and averaging the results. The individual weights are compared with the overall average weight. The standard variation for tablets with an average weight of 350 mg is ±5% (equation 1).
Friability
We used a Roche friabilator to grind ten pills into fine powder for four minutes at 25 rpm. They were removed, dedusted, and weighed again. The formula for calculating the percentage of friability in the tablets (equation 2) is [18]:
where % F is percentage friability, W is the initial weight of the tablet, and Wt is the final weight of tablets after revolutions.
Hardness
The average of the three pills taken from each formulation was used in the study. As a result, the Inweka hardness tester is used to determine how hard each tablet is to the touch. It is measured in kilograms.
Thickness and diameter
Mitutoyo micrometer screw gauge is used to measure tablet thickness and diameter. Each formulation has an average of five pills taken. Millimeter (mm) is the unit of measurement.
Uniformity of drug content
Five pills were ground to powder at random to determine if the drug content was homogeneous. When the drug is dissolved for 5 hours with intermittent shaking, it dilutes to 100 ml of buffer and stores at room temperature. Filtration removes insoluble residue by diluting 1 ml of the filtrate to 10 ml with buffer. We decided to measure the absorbance at its highest using a U.V. visible spectrophotometer. The trials were repeated three times for each formulation, and the mean data was recorded for each.
Drug content was calculated using the following equation (3):
Drug-excipient Compatibility Studies
Fourier transforms infrared spectroscopy (FT-IR)
Drug-excipient interaction studies were conducted to assess the drug's integrity and compatibility in the formulation. Fourier transforms infrared FT-IR spectroscopy was used to examine the pure medication and optimized formulations. FT-IR spectra of pure drug and its formulations were obtained by an "FT-IR Shimadzu 8400S" (Japan) spectrophotometer using the KBr pellet method. The samples were scanned from 400 to 4,000 cm-1 wave number.
Differential scanning calorimetry (DSC)
DSC was carried out on a pure sample of the medication and its powdered form. Calorimetric measurements were performed using high purity alpha-alumina discs as a reference cell. The dynamic scans were taken in a nitrogen atmosphere at the heat rate ± of 10°C min-1. The energy is measured as Joules per kilocalorie [19].
In vitro floating studies [20]
Floating lag time and total floating time were used to measure in vitro buoyancy. A USP dissolving device was used for the test type-II (basket) using 900 ml of 0.1 N HCl buffer solutions at 100 rpm at 37 ± 0.5°C. In this experi-ment, the floating lag and total time are recorded as the time it took for the formulation to reach the surface of the dissolving medium and the time it took for the formulation to remain there (Figure 1).
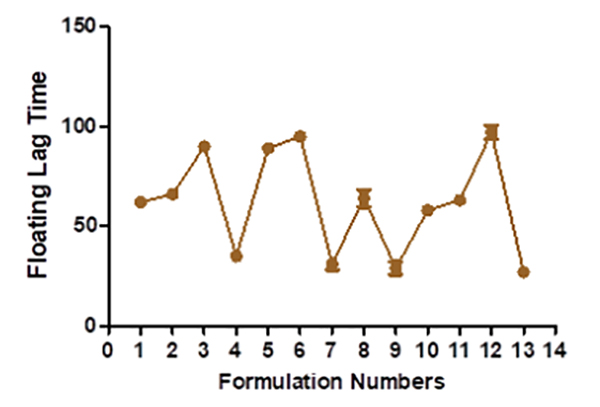 |
Water uptake studies [21]
The swelling of the polymers is based on their propensity to absorb water and expand. A USP dissolving apparatus type-II (basket) was used to dissolve the tablet in pH 1.2 hydrochloric acid buffer at 100 revolutions per minute to conduct a water absorption study (RPM). The medium was maintained at 37 ± 0.5°C throughout the study. Regularly, the tablets were taken out, weighed, and blotted to remove any extra water before being put back in. Water uptake (W.U.) (equation 4) is used to describe the swell ability of the tablets.
Results & Discussion
The hardness of floating tablets ranged from 4.1 ± 1.23 to 6.1 ± 0.306 kg (Figure 2), depending upon the mixture of the polymer used. The tablet formulation's friability ranged from 0.3 ± 0.0063 to 0.59 ± 0.0076%. The manufactured tablet formulation's weight fluctuation is within USP guidelines. The thickness is discovered to be between 4.1 ± 0.48 and 4.2 ± 0.76 mm (Figure 3). The drug content assays ranged from 96.52± 0.37 to 102.03 ± 0.53% (Figure 4).
Potassium bromide dispersion is used to measure the spectra in the solid-state. The FT-IR technology was used to capture the bands. The FT-IR spectral study found that the pure drug and the drug formulation have similar distinctive peaks with slight changes (Table 2). As a result, it was determined that the medication and polymer utilized have no chemical interaction.
Screening for compatibility between drugs and excipients is made more accessible with DSC, which provides a wealth of data on potential interactions in a short period (Table 3). Verapamil hydrochloride and formulation B1 underwent a DSC study. An endothermic peak at 138.25oC, the drug's melting point, maybe seen on its thermogram. The melting point of the medication is found to be 139.53oC in the matrix tablet formulation B1. The medication and excipients have no interaction, according to the analysis of thermograms obtained using DSC [22,23].
At one hour, the B4, B6, and B7 swelled by a large percentage. At the end of 8 hours, B8 exhibited a steady increase in the proportion of swell. The use of karaya gum slows down the water intake in the first hour. During the 8 hours, the levels of B2, B3, and B5 decrease. Sodium bicarbonate concentration does not affect the swelling properties, but lactose concentration in B8 has the most significant effect (p ≥ 0.05) (Figure 5).
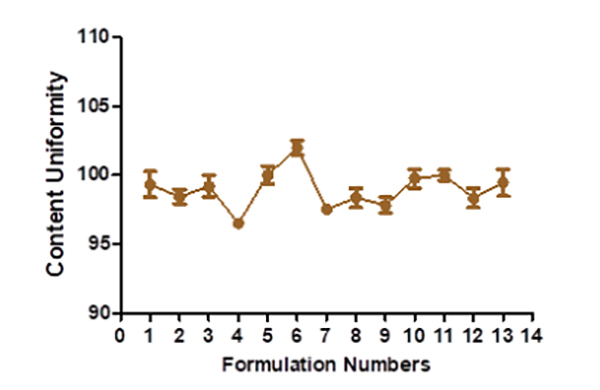 |
Seipmann and Peppas think that the water content of the tablet has a considerable impact on the diffusion of the medication. To understand why this can be the case, we need to understand how water affects the mobility of polymer chains. Polymer chain relaxation occurs with volume increase, resulting in a significant system swell. Higher water content can also indicate faster stomach fluid entry into the tablet, resulting in faster CO2 gas generation and reduced floating time. As a result, the tablet swells faster and more rapidly, increasing the tablet's dimensions and decreasing diffusion rates.
There is more than 75% drug release at 12 hours for each of the following: B1, B5, B6, B9, and B10. At a maximum of 30% in the first hour and for nearly 12 hours, the B1 maintained a steady release of the medication. B8 shows the lowest drug release of all the formulations, which may be due to the substantial gel barrier on the tablet. Other formulations with a more extensive swelling index were shown to have a similar effect on drug diffusion across the gel barrier. B1 had a more excellent lactose content than any of the other samples. An infusion media dispersed into the matrix, leading to drug diffusion and controlled release from tablets (Figures 6 and 7).
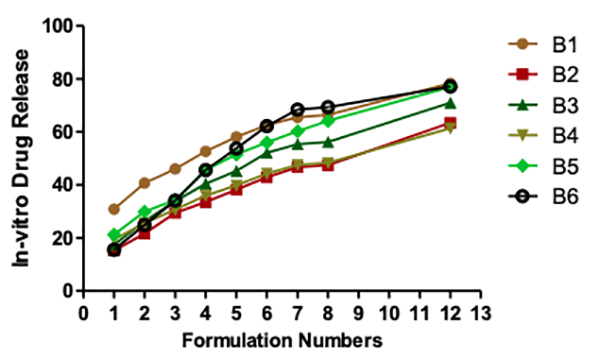 |
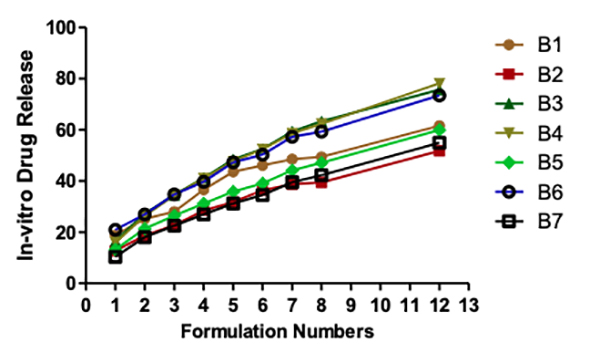 |
To further understand the in vitro drug dissolution profiles, we use various mathematical models, including the Korsmeyer-Peppas equation and Higuchi kinetics, to examine the data. The PCP disso v2.08 software was used to determine the release rates k and n for each model. In order to assess the model's accuracy, correlation coefficients (R2) were used. Table 6.08 lists the R2, k, and n values. Korsmeyer-Peppas and Matrix models were compared using R2 values; the Matrix model was found to have the best R2 values, while Korsmeyer-Peppas had the best R2 values for the other models. There is a wide range in the diffusion exponent. Fickian release was observed in B1, B4, and B7, while the non-Fickian or anomalous release was observed in other formulations. With the Fickian release, B4 and B7 are the best-fitting matrix models; with the non-Fickian release, B5 and B12 are the best-fitting matrix models. Fickian diffusion is employed when the value of n in Korsmeyer-Peppas is 0.5 or less, whereas diffusion with n values between 0.5 and 1 is used for anomalous or non-Fickian releases. When the release mechanism is unclear or numerous types of release phenomena are possible, this model is utilized to explore the release of pharmacological polymeric dosage forms. Fick's laws are the foundation of diffusion, which describes the macroscopic transit of molecules along a concentration gradient.
The optimized formulation B1 was subjected to stability testing to determine the effect of formulation additions on both the chemical and biological stability of the medicine. It was tested for 12 months at 25oC/60 percent R.H., 30oC/65 percent R.H., and 40oC/75 percent R.H. During the course of the study, neither the external appearance nor the pharmacological content changed appreciably. Table 5 provides the results of drug content determination during the period when the stability tests were conducted. Long-term and accelerated storage data were analyzed using Sigmaplot 12.0 software, as was the 95 percent confidence interval. According to the findings, the changes in the parameters evaluated were minor and insignificant.
Conclusion
Direct compression was used to make the tablets. They met pharmacopeial standards for floating tablet technology. More than 8 hours later, the tablets were still floating. After 8 hours, all of the produced formulations have reached complete swelling. Therefore, the percentage of swelling was calculated at that point. More than 75% of the medication was released after 12 hours in F1, F5, F6, F9, and F10. Maximum 30 percent drug release occurred in one hour and continued for over 12 hours with F1. Formulation F1 is deemed the most optimal based on the results of the in vitro testing.
References
- Sarawade A, Ratnaparkhi MP, Chaudhari S. Floating drug delivery system: An overview. Int. J. Res. Dev. Pharm. Life Sci., 2014, 3(5), 1106-1115.
- Bruck SD in Controlled Drug Delivery – Clinical Applications, CRC Press, Boca Raton, 2000, vol. 22, pp 1-15.
- Janseen GM, Robinson JR in Modern Pharmaceutics, 4th Edition, eds. Banker GS, Rhodes CT, 2002, ch. 15.
- Gennaro AR, Remington JP in Remington: The Science and Practice of Pharmacy, 20th Edition, Lippinkott Wilkins & Wilkins, Baltimore, 2000, vol. 1, pp 903-930.
- Chien YW in Novel Drug Delivery Systems, 2nd Edition, CRC Press, pp 1-11.
- Tahmasebi S, El‐Esawi MA, Mahmoud ZH, Timoshin A, Valizadeh H, Roshangar L, Varshoch M, Vaez A, Aslani S, Navashenaq JG, Aghebati‐Maleki L, Ahmadi M. Immunomodulatory effects of nanocurcumin on Th17 cell responses in mild and severe COVID‐19 patients. J. Cell. Physiol., 2020, 236(7), 5325-5338.
- Sharaf HK, Salman S, Abdulateef MH, Magizov RR, Troitskii VI, Mahmoud ZH, Mukhutdinov RH, Mohanty H. Role of initial stored energy on hydrogen microalloying of ZrCoAl (Nb) bulk metallic glasses. Appl. Phys. A, 2021, 127(1), 1-7.
- Mahmoud ZH, Barazandeh H, Mostafavi SM, Ershov K, Goncharov A, Kuznetsov AS, Kravchenko OD, Zhu Y. Identification of rejuvenation and relaxation regions in a Zr-based metallic glass induced by laser shock peening. J. Mater. Res. Technol., 2021, 11, 2015-2020.
- Bahadoran A, Jabarabadi MK, Mahmood ZH, Bokov D, Janani BJ, Fakhri A. Quick and sensitive colorimetric detection of amino acid with functionalized-silver/copper nanoparticles in the presence of cross linker, and bacteria detection by using DNA-template nanoparticles as peroxidase activity. Spectrochim. Acta, Part A, 2022, 268, 120636.
- Mahmoud ZH, Al-Bayati RA, Khadom AA. Synthesis and supercapacitor performance of polyaniline-titanium dioxide-samarium oxide (PANI/TiO2-Sm2O3) nanocomposite. Chem. Pap., 2022, 76(3), 1401-1412.
- Raya I, Widjaja G, Hachem K, Rodin MN, Ahmed AA, Kadhim MM, Mustafa YF, Mahmood ZH, Aravindhan S. MnCo2O4/Co3O4 nanocomposites: Microwave-assisted synthesis, characterization and photocatalytic performance. J. Nanostruct., 2021, 11(4), 728-735.
- Suryatna A, Raya I, Thangavelu L, Alhachami FR, Kadhim MM, Altimari US, Mahmoud ZH, Mustafa YF, Kianfar E. A review of high-energy density lithium-air battery technology: Investigating the effect of oxides and nanocatalysts. J. Chem., 2022, 2022.
- Kaduim D, Mahmoud ZH, Mousa F. Green biosynthesis of iron oxide nanoparticles and testing their inhibitory efficacy against some pathogens. Asian J. Water, Environ. Pollut., 2021, 18(4), 119-123.
- Mahmoud ZH, Al-Bayati RA, Khadom AA. The efficacy of samarium loaded titanium dioxide (Sm: TiO2) for enhanced photocatalytic removal of rhodamine B dye in natural sunlight exposure. J. Mol. Struct., 2022, 1253, 132267.
- Raya I, Widjaja G, Mahmood ZH, Kadhim AJ, Vladimirovich KO, Mustafa YF, Kadhim MM, Mahmudiono T, Husein I, Kafi-Ahmadi L. Kinetic, isotherm, and thermodynamic studies on Cr (VI) adsorption using cellulose acetate/graphene oxide composite nanofibers. Appl. Phys. A, 2022, 128(2), 1-9.
- Brahmankar DM, Jaiswal SB in Biopharmaceutics and Pharmacokinetics, p 348.
- Oudah KH, Najm MA, Samir N, Serya RA, Abouzid KA. Design, synthesis and molecular docking of novel pyrazolo [1,5-a][1,3,5]triazine derivatives as CDK2 inhibitors. Bioorg. Chem., 2019, 92, 103239.
- Oudah KH, Najm MA, Roomi AB, Al-saidy HA, Awadallah FM. The recent progress of sulfonamide in medicinal chemistry. Syst. Rev. Pharm., 2020, 11(12), 1473-1477.
- Najm MA, Abd-Alrassol KS, Qasim QA, Hussein HH, Al-Salman HN. Spectrophotometric determination of folic acid using 1,10-phenanthroline materials with ninhydrin reagent. Mater. Today: Proc., 2021 Nov 12.
- Al‑Salman HNK, Ali ET, Jabir M, Sulaiman GM, Al‑Jadaan SAS. 2‑Benzhydrylsulfinyl‑N‑hydroxyacetamide‑Na extracted from fig as a novel cytotoxic and apoptosis inducer in SKOV‑3 and AMJ‑13 cell lines via P53 and caspase‑8 pathway. Eur. Food Res. Technol., 2020, 246, 1591-1608.
- Alassadi EAS, Jasim EQ, AL-Salman HNK, Mosa MN. A comparative study of an in vitro release patterns of ceftaroline fosamil from chemically-prepared coated hydroxyapatite nanoparticles. Syst. Rev. Pharm., 2020, 11(3), 797-805.
- Fayadh RH, AL-Salman HNK, Hussein HH. A quick, simple, and specific stability indicating RP-HPLC technique for determining valsartan in pharmaceuticals was developed and the validated. Ann. R.S.C.B., 2021, 25(4), 16553-16570.
- Ahmed GS, Abdul Jabbar AS, Jeber MA, Al-Salman HNK, Shari FH, Qasim QA, Hussein HH. Effect of COVID-19 on cancer: With special references to liver cancer. Int. J. Pharm. Res., 2020, 12(4), 3861-3867.
- Pahwa R, Saini N, Kumar V, Kohli K. Chitosan-based gastro retentive floating drug delivery technology: An updated review. Expert Opin. Drug Delivery, 2012, 9(5), 525-539.
|