|
|
|
The Chemist Volume 88 | Number 1 |

|
|
|
Preparation of Zinc and Calcium Silicate Modified
Fly Ash/
Styrene Butadiene Rubber Composites
|
|
Abstract: The present work is focused on the effect of surface modified fly ash as reinforcing filler on the cure, morphology and crystalline nature, thermal, flame and mechanical properties of styrene butadiene rubber (SBR) composites. The surface modification of fly ash was monitored by FTIR and XRD studies. The processing characteristics such as cure and scorch time of SBR decreases with increase in concentration of fly ash, but show an enhancement in maximum torque up to 30 parts per hundred rubber (phr) of filler. The dispersion of fly ash in the polymer was analyzed by SEM and XRD. Mechanical properties such as tensile strength, modulus, tear resistance, hardness and heat build-up of the vulcanizate increases whereas the resilience and elongation at break decreases with loading of fillers. Chemically modified fly ash imparts higher mechanical properties than unmodified fly ash/SBR composites. The thermal and flame resistance of the composite increases with increase in concentration of fillers.
Key Words: SBR, modified fly ash, mechanical properties, thermal stability, flame retardancy.
|
|
|
Introduction
Particulate filled polymer composites are becoming attractive because of their wide applications and low cost. Reinforcement of elastomeric compounds enhances mechanical properties by the addition of inorganic fillers, which is one of the most important phenomena in material science and technology. Incorporating inorganic mineral fillers into elastomers improves various physical properties of the materials, such as mechanical strength, modulus, hardness, etc. In general the mechanical properties of particulate filled polymer composites depend strongly on size, shape and distribution of filler particles in the polymer matrix and extent of interfacial bonding between the filler and matrix [1-3]. Studies of mineral fillers such as talc, kaolin, mica and calcium carbonate are relatively well known to enhance the stiffness and strength of filled rubber. Of more than 100 different types of inorganic/organic reinforcing materials researched and reported in the literature, only a few fillers like carbon black and silica have been commercialized and used extensively [4].
Fly ash (FA) is a waste mineral product of thermal power stations. Environmental problems due to fly ash is a major concern. There are many conventional ways for using the waste fly ash in construction and agricultural sectors to reduce environmental pollution [5]. However, the utilization of fly ash is very low percentage, the remainder being disposed of in landfills, possibly causing serious environmental problems [6,7]. Recently, new ways of utilizing fly ash have been explored to protect the environment and provide useful ways for its disposal.
Styrene butadiene rubber (SBR) find important application in many industries related to tire, hose, cable, health care products, and so on, owing to their excellent abrasion resistance and elastic nature. However, SBR has poor tensile strength and flammability, so great care must be taken in selecting an appropriate elastomer for various applications that required performance and durability. The strength and flame resistance of SBR can be improved by the addition of fly ash particles. A significant problem in fly ash filled system is caused by the poor adhesion and the non-uniform dispersion of filler in the polymer matrix. Surface modification of fly ash by suitable methods can overcome these problems by enhancing surface interactions between the polymer and inorganic fillers. The main objectives of the work are, (1) to prepare zinc and calcium silicate groups on the surface of waste fly ash particles, (2) to prepare modified and unmodified fly ash incorporated SBR composite, (3) to study the processability and mechanical properties of the fabricated polymer composite and (4) to analyze the effect of loading of zinc and calcium silicate modified fly ash on the thermal stability and flame retarding behavior of SBR composites.
Experimental
Materials
Fly ash (FA) (Composition: 63 wt% SiO2, 30 wt%Al2O3, 3.5 wt% MgO, 2.5wt% CaO, 1wt% TiO2 and 0.65 wt %K2O; specific gravity (2.08 g/cm3) was obtained from thermal power station, Deepnagar, Bhusaval, Maharashtra, India. SBR -1502 grade was obtained from Synthetics & Chemicals Ltd, U.P., India. ZnO, stearic acid, TDQ, CBS, processing oil, sulfur, ZnCl2, NaOH, Ca(OH)2 were obtained from local chemical suppliers.
Methods
Surface Modification of Fly Ash
- Preparation of Zinc Silicate Modified Fly Ash
Zinc chloride was dissolved in distilled water followed by adding sodium hydroxide solution (1:1 molar ratio) and stirring the mixture for 15 minutes. The precipitated zinc hydroxide is then washed several times with water to remove the byproduct (NaCl) and any un-reacted zinc chloride. Fly ash was then added to the zinc hydroxide precipitate and stirring was continued for 2 hrs at 800C. The reaction between zinc hydroxide and silanol unit present in fly ash particles leads to the formation of zinc silicate on surface of fly ash.
- Calcium Silicate Modification on Zinc Silicate Coated Fly Ash
Calcium silicate is coated on the surface of zinc modified fly ash by the addition of calcium hydroxide solution (Ca(OH)2 in 200 ml water ) with a ratio of zinc fly ash / Ca(OH)2 of 5:2 and the resulting slurry was refluxed for 4 hrs at 1000C. The reaction between calcium hydroxide with free silanol group present in the surface of zinc silica modified fly ash leads to the formation of calcium silicate on the surface of fly ash. The zinc silicate and calcium silicate coated fly ash (ZnCa FA) was then dried at 1000C.
- Preparation of Modified and Unmodified SBR/Fly Ash Composites
Composites were prepared by mixing SBR with different amounts of modified and unmodified fly ash in a two-roll mixing mill with a friction ratio of 1:1.4, as per ASTM D15-627. The basic formulation used in the study is given in Table 1.
Table 1: Recipe for chemically modified and unmodified fly ash filled SBR composites.
|
|
Ingredients |
Parts per hundred rubber (phr) |
|
|
SBR |
100 |
Stearic acid |
2 |
Zinc oxide |
5.0 |
TDQ |
1.0 |
CBS |
1.8 |
Processing oil |
1 wt% of fillers |
Fly ash |
10, 20, 30 and 40 |
ZnCa silicate modified fly ash |
10, 20, 30 and 40 |
Sulphur |
2.2 |
|
|
Characterization
Cure characteristics of modified and unmodified fly ash/SBR composites were studied using a Monsanto Rheometer R-100 at 1500C according to ASTM D-2705. The compounds were vulcanized to their respective cure time in a hydraulic press at 1500C and a pressure of 4.4 Pascal. The different physical properties such as tensile strength, tear resistance, hardness, resilience and heat buildup were analyzed, as per the relevant ASTM standard.
Result & Discussion
FTIR Characterization
FTIR spectra of fly ash and zinc/calcium silicate modified fly ash are shown in Figure 1. It can be seen that the characteristic OH vibration of fly ash appeared at 3516 and 3446 cm-1. In the case of zinc and calcium coated fly ash, the OH vibration appeared only at 3523 cm-1; this is due to the intermolecular interaction between silanol group of fly ash with the hydroxides of zinc and calcium, which leads to the formation of zinc and calcium silicate on fly ash particles. It is evident from the figure that the characteristic absorption band of the OH bond of modified fly ash is shifted to higher frequencies (i.e., 3516 to 3523 cm-1), indicating the chemical modification of fly ash particles. Moreover, the reaction between zinc and calcium hydroxide with silica unit of fly ash produces a new absorption band at 741 cm-1, which confirms the formation of zinc and calcium silicate on the surfaces of fly ash particles.

Fig 1. FTIR spectra of fly ash and zinc and calcium silicate modified fly ash |
XRD Pattern of Surface Modified Fly Ash Particles
XRD is used to analyze the chemical composition and crystal structure of the prepared fly ash particles. Fly ash modified with zinc and calcium silicate showed various diffraction patterns in the XRD spectra indicating the crystalline nature of the samples (Figure 2). It is observed that chemically modified fly ash shows the diffraction pattern of quartz at 2θ ~ 40.60, 46.40 and 55.60 along with the mullite XRD peak at 2θ ~ 30.80 and 35.40 [8]. The characteristic peak of mullite is stronger; whereas, the characteristic peaks of quartz (SiO2) become weaker, which indicate that the modification is mainly taking place on the silanol group present in the surface of fly ash particles. The reaction between hydroxides of zinc and calcium with the silanol or alumina group present on the surface of fly ash particles lead to several silicate and aluminate components such as Zn4Si2O7(OH)2.(H2O), ZnSiO4 Ca3Si3O9.H20, CaAl2(SiO3)4.2H2O, Ca5Al6O14 [9]. These inorganic components that adhere to the surface of fly ash particles affect its surface morphology.
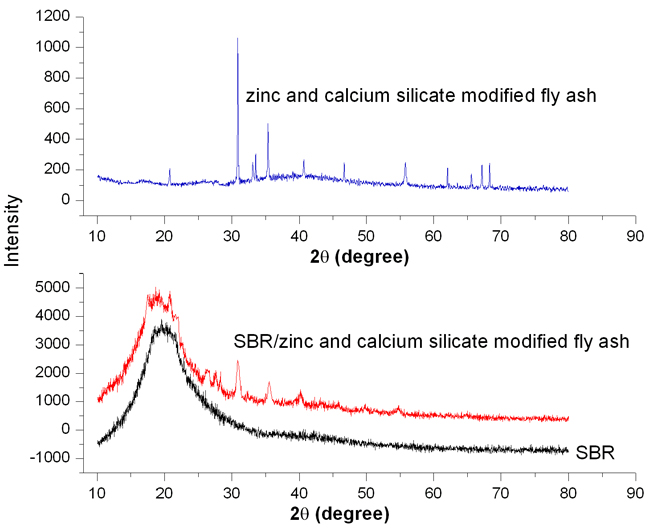
Fig 2. XRD pattern of chemically modified fly ash, SBR and ZnCa-fly ash filled SBR. |
XRD Studies of SBR/ZnCa-Fly Ash Composites
The XRD pattern of SBR and SBR with 30 phr of ZnCa-fly ash composite is given in Figure 2. It is observed that pure SBR has a broad diffraction peak at about 2θ ~ 200, indicating its amorphous nature. However, the XRD pattern of SBR/ZnCa-fly ash composite exhibit few peaks of modified fly ash along with the amorphous peak of SBR. The slight crystalline nature of the composite indicates that filler particles are uniformly distributed into the macromole chain of SBR, which is due to the strong interaction between modified fly ash and the polymer.
Morphology of SBR/ZnCa-Fly Ash Composites
SEM images of SBR with zinc and calcium silicate coated fly ash are shown in Figure 3. It can be observed that at lower concentration of fillers (10 phr), the fly ash particles are unequally distributed within the polymer matrix. As the concentration of filler increased to 30 phr, the fly ash particles are found to be uniformly dispersed in the polymer with a smooth surface. Modifying fly ash with zinc and calcium hydroxide, leading to the formation of zinc and calcium silicate, is the main reason for getting the uniform morphology. The smooth and spherical structure of fly ash particles after the chemical modification can be helpful in increasing the effective surface area for interacting with the polymer chains. However, as the concentration of fly ash increased to 40 phr, the surface of the composite becomes mottled, indicating the agglomeration of fly ash particles.
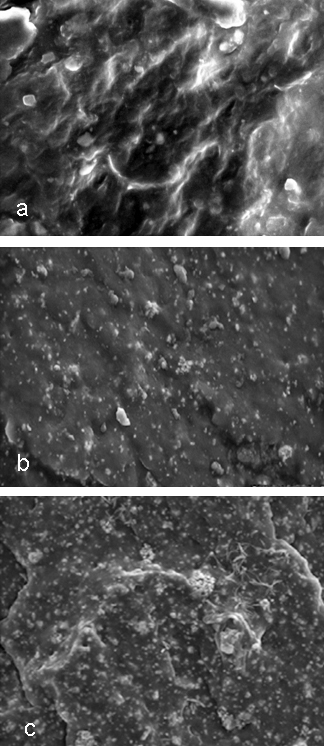
Fig 3. SEM picture of (a) 10 phr (b) 30 phr (c) 40 phr of ZnCa-fly ash filled SBR. |
Processing Characteristics of SBR/ZnCa-Fly Ash Composites
The cure characteristic of SBR with various dosages of modified and unmodified fly ash at 1500C is given in Table 2. The cure time and scorch time decreases not only with the loading of fillers, but also with the chemical modification of fly ash particles. The reduction in optimum cure time is due to the effect of increased heat conductivity of different metal particles present in fly ash. Moreover, the surface modification in fly ash particles reduces the polarity of the filler particles (silanol group undergoes reaction with zinc and calcium hydroxide), which is responsible for the better processability of modified fly ash/SBR composite. It is also evident from the table that the maximum torque increased with increase in concentration of filler up to 30 phr. This is due to the strong adhesion between the filler and the polymer. The minimum torque value is found to be lower for an unfilled sample, which is due to the poor reinforcement effect of the matrix. It can be inferred that the processability of the composite is further enhanced by the use of chemically modified fly ash particles as compared to unmodified fly ash/SBR composite.
Table 2: Cure characteristics of modified and unmodified fly ash filled SBR composites. |
|
Properties |
SBR/ZnCa-flyash (phr) |
|
0 |
10 |
20 |
30 |
40 |
|
|
Maximum torque (dNm) |
30 |
32
(29)*
|
34.5
(31.2) |
36.6
(34) |
35.5
(32.5) |
Minimum torque (dNm) |
7 |
9
(8)
|
10
(9) |
11.5
(10) |
11.7
(10) |
Optimum cure time (min) |
13 |
11
(13)
|
10
(12) |
9
(11) |
8
(10) |
Scorch time (min) |
3.0 |
2.75
(2.5)
|
2.5
(2.25) |
2.25
(2) |
2.0
(1.75) |
|
*Values in parentheses indicate unmodified fly ash composites |
|
Mechanical Properties
The influence of fly ash and ZnCa-fly ash on the physical properties of SBR is given in Table 3. The modulus at 200% elongation increases with increasing the loading of fly ash particles up to 30 phr. The strong adhesion between fly ash and SBR is responsible for the modulus enhancement. The tensile strength of the composite increased with the increase in loading of filler up to 30 phr. Further addition of filler results in a slight decrease in mechanical strength, due to the agglomeration of filler particles. As the loading of filler in the polymer increases, elongation at break decreases and the reduction in elongation at break are considered as the criteria for the reinforcement of fillers [10,11]. Like tensile strength, the tear resistance value increases with increase in loading of filler up to 30 phr (Table 3). This means that the more uniform dispersion of filler in SBR is due to the large interfacial area of contact resulting from chemical modification of fly ash particles [12]. Resilience showed a continuous decrease with loading of fillers whereas the hardness increases. Hardness of vulcanizate is usually increased by the addition of reinforcing fillers. Also, the increase in hardness is usually related to the high tensile modulus and the increasing amount of fly ash particles in the polymer matrix. It is also observed from the table that the modified fly ash composite exhibit better mechanical properties than unmodified fly ash filled SBR compounds.
Table 3: Mechanical properties of FA and ZnCa-fly ash/SBR composites. |
|
Properties |
Fly ash particles (phr) |
|
0 |
10 |
20 |
30 |
40 |
|
|
Tensile
strength (MPa) |
2.0 |
2.9
(2.3)* |
3.5
(3) |
5.2
(4) |
5.1
(3.9) |
Tear
strength (kN/m) |
33 |
35
(33) |
39
(37) |
42
(40) |
40
(37) |
Modulus,
200 % (Mpa) |
1.1 |
2.0
(1.3) |
2.8
(2) |
3.4
(2.5) |
3.1
(2.4) |
Elongation
at break (%) |
430 |
420
(397) |
390
(365) |
380
(350) |
365
(335) |
Hardness
(Shore A) |
32 |
34
(34) |
36
(35) |
39
(38) |
42
(41) |
Resilience (%) |
39.0 |
37
(36) |
35
(34) |
33
(32) |
31
(30) |
Heat build-up |
15 |
17
(17) |
20
(19) |
23
(22) |
26
(24) |
LOI |
16 |
18 |
20.4 |
22.8 |
24.2 |
|
*Values in parentheses denote unmodified fly ash composites |
|
Thermogravimetric Analysis (TGA)
Figure 4 shows the thermogravimetric curves of SBR and SBR with 20 phr and 40 phr of modified fly ash particles. All of the samples undergo single stage decomposition. SBR decomposes at 3650C, while the composite with 20 phr of composite decomposes at 3760C (whereas 40 phr composite decomposes at 3850C). The higher thermal stability of the composites is due to the interaction of fly ash with the macromolecular chain SBR. It can also be seen from the figure that the final residue (char) of SBR is about 10%, compound with 30 phr, exhibit a char residue at 14%. It is already reported that the final char residue resulting after the thermal decomposition is the measure of the flame resistance of a material [13]. The increase in char residue with the modified fly ash particles clearly proves that the flame resistance of the composite increases with the addition of fillers. However, this will be explained more specifically with the help of LOI values.
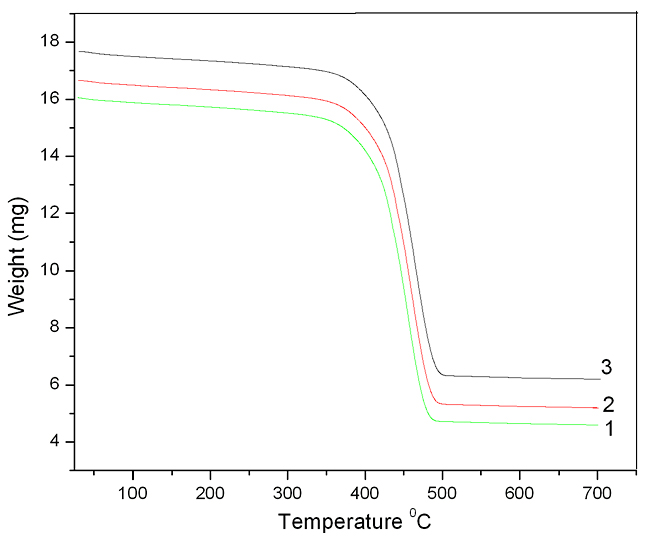
Fig 4. TGA curve of (1) SBR (2) 20 phr (3) 40 phr fly ash filled SBR. |
Effect of ZnCa-Fly Ash on the Flame Retardency Behavior
The limiting oxygen index (LOI) value is expressed as a number indicating the percentage of oxygen needed for the self-combustion of a material. LOI is the direct measure of the flame resistance of a polymeric material. The LOI value of SBR with 0, 10, 20, 30, 40 phr of zinc and calcium coated fly ash composites are given in Table 3. It is clear from the table that the flame resistance of composite is higher than that of pure SBR and the values increases with the increase in concentration of fillers. The pure SBR has LOI value of 16, whereas composite with 20 phr exhibit a LOI value of 20.4. The enhanced interaction between fly ash and the polymer may improve the flame retardency of composite by the improved dispersion level and heat flow resistance. Thus, dispersion of fly ash particles in the polymer is an important criterion for obtaining improved flame retardency [14]. If metal oxide particles, such as Al2O3, Fe2O3, K2O3, SiO3, etc., are present in the surface of fly ash particles, the carbonization of polymer is reduced forming more protective char layer that leads to improved flame retardency behavior of the composite.
Conclusions
Zinc and calcium silicate modified fly ash was successfully prepared and characterized by FTIR and XRD measurements. Unmodified and modified fly ash particles incorporated in SBR composite were prepared by adding various loading of fly ash in a two-roll mixing mill. The cure characteristics, morphology, crystalline nature, mechanical properties, thermal stability and flame resistance of fly ash filled SBR was studied. Cure characteristics of the composite showed that the optimum cure time and scorch time decrease with loading of filler, whereas the rheometric torque increased with loading of fly ash particles. Surface modification on fly ash improved the processability of SBR composite as compared to unmodified fly ash/SBR samples. The XRD patterns of modified fly ash/SBR composite showed few peaks of modified fly ash along with the amorphous peak of SBR. SEM images showed that the composite with 30 phr fly ash filled SBR showed better dispersion of filler. Thermal stability and flame resistance of the composite was higher than that of pure SBR. The mechanical properties such as tensile strength modulus and tear resistance were found to be increases with increase in loading of fly ash particles up to 30 phr, and thereafter the properties decreased. Hardness increases where as the resilience decreases with increase in loading of fillers. Surface modified fly ash was able to give an enhancement in cure and mechanical properties of SBR composites in comparison with the modified fly ash SBR composite.
References
- Mishra S, Shimpi NG. Polym. Plast. Technol. Eng., 2007, 47, 72-81.
- Nath DCD, Bandyopadhyay S, Yu A, Blackburn D, White C. J. Mater. Sci., 2010, 45, 1354-1360.
- Ramesan MT, Premalatha CK, Alex R. Plast. Rubber. Comp., 2001, 30, 355-362.
- Mohapatra R, Rao JR. J. Chem. Tech. Biotechnol., 2001, 76, 9-26.
- Horiuchi S, Kawaguchi M, Yasuhara K. J. Hazard. Mater., 2000, 76, 301-337.
- Ramesan MT. Pet. Sci. Technol., 2014, 32, 1775-1783.
- Thongsang S, Sombatsompop N, Ansarifar A. Polym. Adv. Technol., 2008, 19, 1296-1304.
- Hill RJ, Gibbs GV, Craig JR, Ross FK, Williams JM. Z. Kristallogr., 1977, 146, 241-259.
- Lu P, Li Q, Zhai J. J. Amer. Ceramic Soc., 2008, 91, 955-964.
- Ramesan MT, Nihmath A, Francis J. AIP Conf. Proc., 2013, 1536, 255-256.
- Ramesan MT. J. Appl. Polym. Sci., 2014, 131, 3681-3689.
- Ramesan MT. J. Elast. Plast., 2014, 46, 303-324.
- Seema A, Kutty SKN. Int. J. Polymer. Mater., 2006, 55, 25-35.
- Ramesan MT. Polym. Eng. Sci., 2014, 54, 438-445.
|